WHAT IS REGENERATIVE BRAKING?
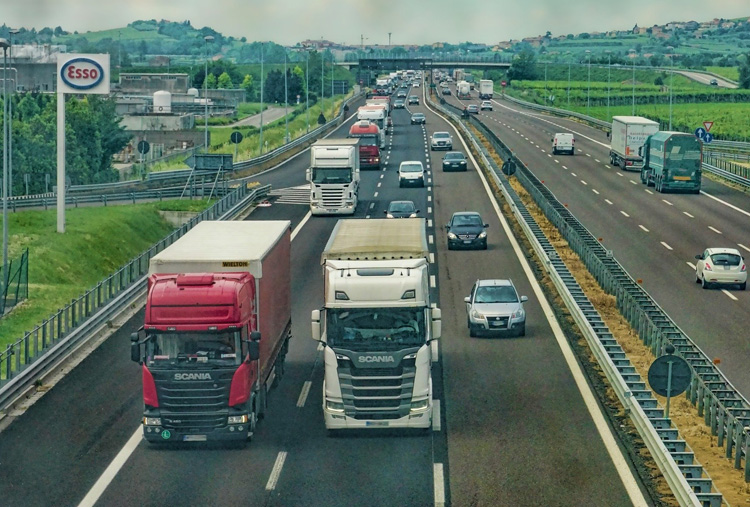
Imagine if you could reclaim some of the energy you lose throughout the day, without needing to rest. Did you know that electric vehicles (EVs) are able to do this through regenerative braking? An efficient way to reuse some of the energy lost as heat when a vehicle brakes, regenerative braking supports higher efficiency and the ability to travel further on a single charge.
When the driver steps on the brake pedal of a vehicle, hydraulic fluid pushes the brake pads against brake discs on each wheel. This friction slows down the vehicle, but the process also creates heat and wears away the material on the pads and discs over time.
Regenerative braking uses the excess kinetic energy to recharge an EV’s battery. It is able to do this because the electric motor in an EV can run in two directions: one, using the electrical energy, to drive the wheels and move the car, and the other, using the excess kinetic energy, to recharge the battery.
When the driver lifts their foot off the accelerator pedal and steps on the brake, the motor starts to resist the vehicle’s motion, “swapping direction”, and begins putting energy back into the battery. As a result, regenerative braking uses the EV’s motor as a generator to convert lost kinetic energy into stored energy in the battery.
A NECESSITY
The Competition and Market Authority has warned the UK government that, ahead of the petrol ban in 2030, more electric charging points must be established to make EV charging easier for road users. As it stands, there are only 25,000 public charging points in the UK. This needs to increase by tenfold to ensure EV success from 2030 onwards.
While not fundamentally an element of EV charging infrastructure, regenerative braking provides a way of making EVs more efficient by increasing the number of miles completed without charging the battery. Furthermore, the process can help make the charging less reliant on electricity from the National Grid. By reducing the frequency of charging and amount of electricity needed to recharge batteries, regenerative braking can make the entire charging process more energy efficient.
A HELPING HAND
However, regenerative braking cannot act alone. To work effectively, other technologies are needed to make the process safe and effective. If the car battery is already full or there is a failure, regenerative braking cannot happen as the excess energy has nowhere to go and must be dispelled safely. If not dissipated, it won’t be possible to slow down the vehicle. To prevent this from happening, resistors are used to collect excess energy and dissipate it safely.
Cressall’s EV2 resistor converts excess electricity into heat that can be dissipated or used in other parts of the vehicle, such as to heat the cabin, the batteries or even the fuel cell. The EV2 is a lightweight, compact resistor which manages to transfer this heat into the cooling water or glycol mix, which is already used in the cooling or heating system for different vehicle components. Cooling is achieved by pumping cold coolant liquid, which comes into one end of the unit and absorbs the heat through thermal conductivity and convection. It can then be pumped through a radiator located away from unit and cooled again to reach the starting temperature.
While it may not be possible for humans to regain lost energy without taking time out to recharge, regenerative braking enables EVs to use excess energy to work more efficiently. With the help of resistors, EV users can benefit from a longer battery life, helping to drive EV efficiency forward and ensuring safe driving in any conditions.
CR473